-
What is the relationship between fuel nitrogen and NOx emissions?
Date posted:
-
-
Post Author
espadmin
1. Introduction
The nitrogen contained in some fuels takes a very active part in forming [GLOSS]nitrogen oxides[/GLOSS] via the so-called [GLOSS]fuel NO[/GLOSS]mechanism. The importance of this mechanism relative to other NOx formation routes depends, among other things, on the nitrogen content of the fuel. This NOx formation mechanism is the subject of the present combustion file. Other mechanisms are outlined in CF66, and described in detail in other separate combustion files (CF40, CF42, and CF180).
2. Nitrogen content of common fuels
The parameters that mainly affect fuel NO formation are the amount of nitrogen present in the fuel and the [GLOSS]air-fuel stoichiometry[/GLOSS].
The amounts of nitrogen found in fuels vary significantly: as an example, Table 1 lists the nitrogen contents of a number of common fuels. In [GLOSS]fuel oil[/GLOSS], the concentration of nitrogen typically increases with the oil density, i.e., [GLOSS]heavy fuel oil[/GLOSS] typically have more nitrogen than [GLOSS]light fuel oil[/GLOSS]. For [GLOSS]coal[/GLOSS], the nitrogen content also varies depending on the coal type (CF177), but it usually ranges between 0.5-3% by weight (dry basis). In biomass fuels it usually varies between 0.1 and 0.5 %, whereas, the percentage of nitrogen contained in waste derived fuels depends on the waste composition. However, with a few exceptions such as plastic waste, all of them contain some amount of nitrogen.
Nitrogen is largely organically bound in the fuel, even though in high rank coals some nitrogen may be present in [GLOSS]inorganic[/GLOSS] form as ammonium ions. In coals, a large part of the fuel-nitrogen is bound in [GLOSS]aromatic structures[/GLOSS] such as [GLOSS]pyridine[/GLOSS] (6-ring) and [GLOSS]pyrrole[/GLOSS] (5-ring). In biomass, the nitrogen is also bound in [GLOSS]amino-type structures[/GLOSS], especially within the [GLOSS]proteins[/GLOSS] in [GLOSS]wood[/GLOSS] and [GLOSS]peat[/GLOSS]. Nevertheless, the conversion of fuel nitrogen to NO is nearly independent on the structure where the nitrogen is initially bound.
We additionally note here that inorganically bound nitrogen is often present in[GLOSS]natural gas[/GLOSS], but such nitrogen (N2) does not take part in the fuel NO mechanisms described in this combustion file, instead, it acts via the molecular nitrogen mechanisms (CF40, CF42, CF180).
Fossil fuels |
Biomasses & Waste Derived – Fuels |
||
Coal |
0.5 – 3 |
Wood |
0.1 – 0.5 |
Oil |
<1 |
Straw |
0.5 –1 |
Natural Gas |
0.5 – 20 * |
Sewage Sludge |
~ 1 |
Light fuel oil |
~ 0.2 |
Municipal Solid Waste (MSW) |
1 – 5 |
Heavy fuel oil |
~ 0.5 |
(RDF) and (PDF) ** |
~ 1 |
Peat |
1 – 2 |
Auto shredder residue (ASR) |
~ 0.5 |
Petroleum coke |
~ 3 |
Leather waste |
~ 12 |
Orimulsion |
~ 4 |
Black liquor solids |
0.1 – 0.2 |
*) this nitrogen is in the form of N2 |
|||
**) RDF = Refuse Derived Fuels, and PDF = Packaging Derived fuel
Table 1: Typical values for the nitrogen content of fuels (dry %-wt). Adapted from Zevenhoven and Kilpinen, 2001 3. Fuel NO formation3.1. Pyrolysis[GLOSS]Pyrolysis[/GLOSS] is a thermochemical process where the volatile components ([GLOSS]volatiles[/GLOSS]) of solid fuels are extracted from the solid matrix ([GLOSS]char[/GLOSS]). Any solid fuel undergoes pyrolysis as it is exposed to the elevated temperatures in combustion devices. During pyrolysis, the nitrogen from the fuel is redistributed between the volatile components and the char, with the partitioning determined by the temperature, fuel type, and residence time. In the volatiles, the nitrogen is further partitioned between tar and gas phase components (mainly HCN and NH3). The pyrolysis process described above is followed by a so-called “secondary pyrolysis”, during which both tar and char undergo further decomposition and release part of their nitrogen to the gas phase, mostly as HCN but also NH3. Both, in presence of oxygen, react to form fuel NO. The reactions involved are explained below. After pyrolysis, and if the char is exposed to an oxidising environment, the remaining char nitrogen undergoes heterogeneous oxidation producing NO, N2O. Alternatively, at high temperatures the nitrogen may evolve into light components such as HCN. Once formed, NO may be recycled to N2 by surface reactions on char and soot, and may react with hydrocarbon radicals to form cyanide (HCN) and also N2. Split between volatiles and charThe fraction of nitrogen released with volatiles during pyrolysis increases with: 1. The oxygen content in the parent coal, i.e. with decreasing[GLOSS]coal rank[/GLOSS]. 2. The temperature of pyrolysis or residence time.
Gas phase nitrogen species speciation The gas phase nitrogen species speciation is often of little importance for the NO formation because all species feed principally into the amine radical pool [GLOSS]NHi[/GLOSS] (see Figure 1). The subsequent reactions only depend on the flame conditions, i.e. stoichiometry, temperature, etc. Only under very low temperatures is it considered important, because, under these conditions, HCN also reacts to form considerable amounts of N2O. HCN/NH3 ratio during pyrolysis increases with: 1. the geological age of the fuel: old fuels such as coal release much of the fuel-nitrogen in the form of HCN, whilst “younger” fuels like peat, biomass and wood release most of the fuel as NH3. 2. the O/N ratio of the fuel: the lesser oxygen content compared to nitrogen in the fuel, the higher the HCN/NH3 ratio. 3. the coal rank, which relates to the geological age of the coal (see number 1). the heating rate: at high heating rates HCN is the primary product, whereas at low heating rates NH3 is the dominant product. |
3.2. Oxidation of HCN and NH3
The main reactions describing the behaviour of HCN and NH3 are explained in Figure 1.
The gas-phase fuel-nitrogen reaction sequence is initiated by a rapid and nearly quantitative conversion of the parent fuel-nitrogen compounds to HCN. NH3 is formed from both by direct release from the solid matrix and from the hydrogenation of HCN on the surface of the solid matrix. Both HCN and NH3 react relatively fast to finally form the amine radical pool NHi through the reactions described in Figure 1.
NHi under fuel lean conditions is oxidised to form NO, whilst under fuel rich conditions it reacts with another nitrogen containing species to form N2 (see Figure 1).
At low temperatures (1100-1200 K) as in fluidised bed combustion, about 10 to 50% of the HCN and HiNCO compounds are oxidized homogeneously to laughing gas (N2O) through the following sequence:
HCN + O => NCO + H (1)
NCO + NO => N2O + CO (2)
Above 1200 K no more N2O is formed and NCO reacts to form N2 and NO.
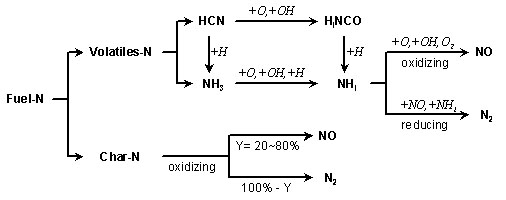
Figure 1: Schematic of oxidation of fuel nitrogen to NO and N2. The “Y” indicates the fraction of N (in mass). From Zevenhoven and Kilpinen, 2001
Reducing NO formation from oxidation of HCN and NH3 can be achieved by arranging “zones” with reducing atmosphere (fuel-rich conditions) in the furnace during the pyrolysis stage. This can be obtained via [GLOSS]air staging[/GLOSS] (see CF128). With this technique, between 10 and 50% of volatilised fuel-N can be converted to N2.
3.3. Oxidation of char-nitrogen
The oxidation mechanism of char-nitrogen includes not only [GLOSS]homogeneous reactions[/GLOSS] and [GLOSS]heterogeneous reactions[/GLOSS] but also mass transfer effects inside the pore system of the char and in the boundary layer surrounding the particle.
Under oxidising conditions char-nitrogen reacts with O2, producing NO, N2O and N2. In reducing conditions (deficit of oxygen), char-nitrogen remains mostly un-reacted. The main char-nitrogen reactions are believed to be:
C(N) + O2 => NO + C(O) (3)
NO + 2Cr => C’(N) + C(O) (4)
C’(N) + C’(N) => N2 + 2Cr (5)
C’(N) + NO => N2 + C(O) (6)
where (…) denotes species on the char surface, ‘(…) the same species but in a different site than (…), and Cr denotes a free carbon site.
The nitrogen contained within the char reacts with O2 producing NO. The NO formed may be significantly re-adsorbed on the char surface by reacting with a free carbon site. Finally, the formed NO may be reduced through recombination of two surface nitrogen species or by reaction of one of them with NO.
At low temperatures as in fluidised bed combustion, considerable amounts of N2O may also be formed. However, N2O can also be reduced to N2 when reacting with char.
Char-NO cannot be directly controlled by air staging since under reducing conditions, char-N mostly remains unreacted. Therefore, in modern boilers, the relative importance of char-NO on the total NO emission increases.
Indirect control is possible because the fraction of N released during pyrolysis increases with increasing temperature/time development. Therefore, at high temperatures less nitrogen remains in the char.
4. When is Fuel NO important compared to other NOx formation mechanisms?
The fuel NO mechanism is a major source of NOx in pulverized coal fired (PCF) combustors. In a standard PCF combustor 80% of NOx is formed from the fuel-N. The remaining portion of NOx is then formed from the atmospheric N2: ~15% via the thermal-NO mechanism (CF40), and ~5% via the prompt-NO mechanism (CF42).
The fuel-N can be considered almost the only source of NOx also in low temperature combustion devices such as fluidised bed combustors (FBC), where the temperature is maintained far below the levels demanded to initiate NOx via the thermal mechanism.
On the contrary, the fuel NOx mechanism is irrelevant in internal combustion engines (e.g., otto engines, diesel engines, and gas turbines) when these devices are operated with conventional fuels like petroleum-derivates and natural gas. This is mainly because the conditions inside engines are strongly favourable for the thermal NO mechanism. Nevertheless, when engines are operated with biomass-derived fuels such as [GLOSS]biodiesel[/GLOSS] or [GLOSS]syngas[/GLOSS] with a higher fuel-N content, then the portion of NOx due to the fuel NO mechanism is usually non-negligible, and sometimes becomes even dominant.
Acknowledgements
We would like to thank Dr. Kilpinen for her good advices and kind support.
Sources
[1] Proceedings of the 6th International Conference on Technologies and Combustion for a Cleaner Environment, Oporto, Portugal, July 9-12, 2001
[2] Zevenhoven R., Kilpinen P., Control of pollutants in flue gases and fuel gases, Picaset Oy, Espoo, ISBN 951-22-5527-8, 2001 (e-book at www.hut.fi/~rzevenho/books.html)