-
How do I model mercury oxidation?
Date posted:
-
-
Post Author
espadmin
1. Background
Mercury emitted from coal combustion is oxidised at relatively lower temperatures in the flue gas stream by the chlorine and bromine species that are also released from the coal combustion process. Oxygen-containing radicals such as O and OH do not play a significant role in mercury oxidation other than to influence the radical pool distribution. The approach to model the oxidation processes is to take the base reaction mechanisms of mercury interaction with chlorine, and the analogous reactions with bromine, then combine these with a simple gas phase chemical kinetic mechanism that describes the chemistry of the flue gas composition, along with mechanisms that define the remaining halogen chemistry. Barring an experimentally determined initial composition, the initial conditions of the flue gas stream in terms of the chlorine, bromine, carbon, hydrogen, nitrogen and oxygen partitioning amongst the possible species, can be estimated based on an equilibrium distribution amongst the constituent species at a defined temperature, pressure, and major stable species composition.
2. Mercury – chlorine – bromine kinetic mechanism
An 8 step mercury – chlorine reaction mechanism as originally proposed by Widmer at al. [1] is given in the following reactions 1 to 8. An analogous set of reactions defines the mercury – bromine chemistry in reactions 9 to 16.
Hg + Cl + M ⇌ HgCl + M (1) Hg + Br + M ⇌ HgBr + M (9)
Hg + Cl2 ⇌ HgCl + Cl (2) Hg + Br2 ⇌ HgBr + Br (10)
Hg + HCl ⇌ HgCl + H (3) Hg + HBr ⇌ HgBr + H (11)
Hg + HOCl ⇌ HgCl + OH (4) Hg + HOBr ⇌ HgBr + OH (12)
HgCl + Cl + M ⇌ HgCl2 + M (5) HgBr + Br + M ⇌ HgBr2 + M (13)
HgCl + Cl2 ⇌ HgCl2 + Cl (6) HgBr + Br2 ⇌ HgBr2 + Br (14)
HgCl + HCl ⇌ HgCl2 + H (7) HgBr + HBr ⇌ HgBr2 + H (15)
HgCl + HOCl ⇌ HgCl2 + OH (8) HgBr + HOBr ⇌ HgBr2 + OH (16)
Rate coefficient parameters
For the mercury-chlorine chemistry, reaction 1, and also the rate of chlorine atom recombination are given by Donohue [2]. Rate coefficients for Reactions 2 to 8 are taken from the theoretical study by Krishnakumar and Helble [3], and the remaining reactions of the mechanism from Roesler et al. [4] are used to describe the chlorine chemistry. For the mercury-bromine chemistry, reaction 9 and bromine atom recombination are again given by Donohue [2]. Niksa et al. [5] give the rate coefficient of reaction 12, Wilcox and Okano [6] provide rate coefficient values for 10, 11, 13 to 16, while Molina et al. [7] define the remaining bromine reactions.
There is much uncertainty concerning the rate coefficients of many of these reactions. Therefore, a valid approach is to apply an optimisation procedure in order to identify both those reactions that are especially important and also to provide optimal values of the reaction Arrhenius parameters. From the work of Clements et al. [8], a [GLOSS]genetic algorithm[/GLOSS] optimisation methodology against lab-based target datasets of mercury oxidation as a function of HCl and HBr concentration is used to optimise the most sensitive mercury and halogen reactions. Those reactions modified from their literature sources are given in the following table.
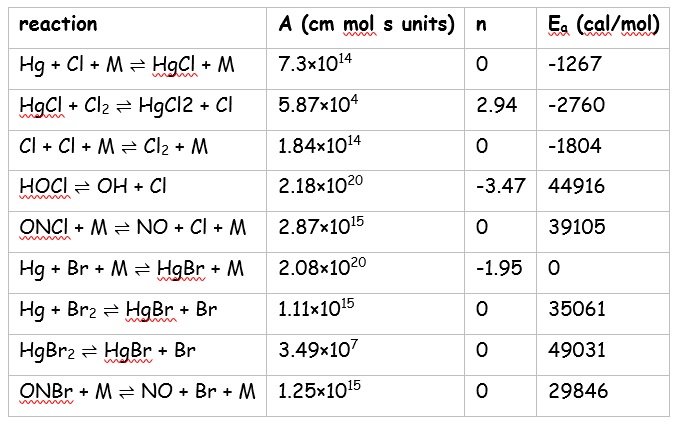
The base C/H/N/O oxidation scheme with which these halogen chemistry reactions are amalgamated is the [GLOSS]GRI-Mech 3.0[/GLOSS][9].
3. Validation
The combination of the reactions described in Section 2, based on GRI-Mech 3.0, with the general halogen chemistry and specific halogen-mercury chemistry, has been validated by Clements et al [8] against experimental datasets produced by Preciado et al. [10]. The capability of the optimised model to predict the experimental results in the case of both HCl and HBr addition, in both air and oxygen (27%) enriched cases is illustrated in the following two figures, showing in general an excellent agreement.
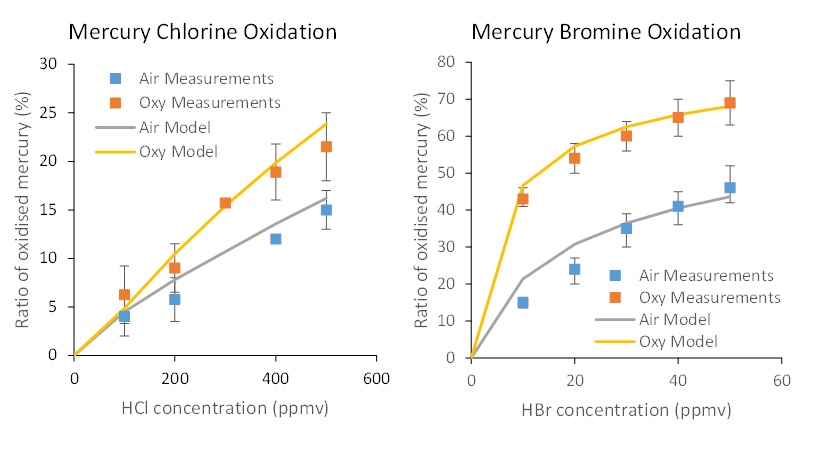
Sources
[1] N.C. Widmer, J. West, J.A. Cole. Thermochemical study of mercury oxidation in utility boiler flue gases. Proc. A&WMA Annual Conference, Salt Lake City, UT, June, 2000.
[2] D.L. Donohoue, Kinetic Studies of the Oxidation Pathways of Gaseous Elemental Mercury, Ph.D. thesis, University of Miami, 2008.
[3] B. Krishnakumar, J.J. Helble, Determination of transition state theory rate constants to describe mercury oxidation in combustion systems mediated by Cl, Cl2, HCl and HOCl, Fuel Processing Technology 94:1 1 2012.
[4] J.F. Roesler, R.A. Yetter, F.L. Dryer, Kinetic interactions of CO, NOx, and HCl emissions in postcombustion gases, Combustion and Flame 100:3 495 1995.
[5] S. Niksa, B. Padak, B. Krishnakumar, C. V. Naik, Process Chemistry of Br Addition to Utility Flue Gas for Hg Emissions Control, Energy & Fuels 24:2 1020 2010.
[6] J. Wilcox, T. Okano, Ab initio-based Mercury Oxidation Kinetics via Bromine at Postcombustion Flue Gas Conditions, Energy & Fuels 25:4 1348 2011.
[7] A. Molina, J.J. Murphy, C.R. Shaddix, L.G. Blevins, The effect of potassium bromide and sodium carbonate on coal char combustion reactivity, Proceedings of the Combustion Institute 30:2 2187 2005.
[8] A.G. Clements, K.J. Hughes, R.T.J. Porter, M. Pourkashanian, Modelling Investigation into mercury oxidation under oxyfuel conditions, Proceedings of the 18th IFRF Members Conference, Freising, Germany, 2015.
[9] G. P. Smith, D. M. Golden, M. Frenklach, N. W. Moriarty, B. Eiteneer, M. Goldenberg, C. T. Bowman, R. K. Hanson, S. Song, W. C. Gardiner, Jr., V. V. Lissianski, and Z. Qin, GRI-Mech 3.0, http://www.me.berkeley.edu/gri_mech/ 1999.
[10] I. Preciado, T.R. Young, G.D. Silcox, Mercury Oxidation by Halogens under Air-Fired and Oxygen-Fired Conditions, Energy & Fuels 28:2 1255 2014.
Acknowledgements
The preparation of this Combustion File was supported by RELCOM; Reliable and Efficient Combustion of Oxygen/Coal/Recycled Flue Gas Mixtures – a project undertaken with the financial support of the European Commission under FP7 Grant Agreement Number 268191.